Monitoring water quality in aquaculture and fish farming
Aquaculture — the farming of fish, crustaceans, mollusks, and aquatic plants — is a major source of food and economic activity worldwide. Regardless of system type (ponds, cages, tanks, raceways, or recirculating aquaculture systems [RAS]), maintaining optimal water quality is crucial for animal health, growth rates, and production efficiency.
Accurate, real-time monitoring of pH, dissolved oxygen (DO), salinity, ammonia, oxidation-reduction potential (ORP), and turbidity ensures optimal environmental conditions and minimizes disease risk and mortality.
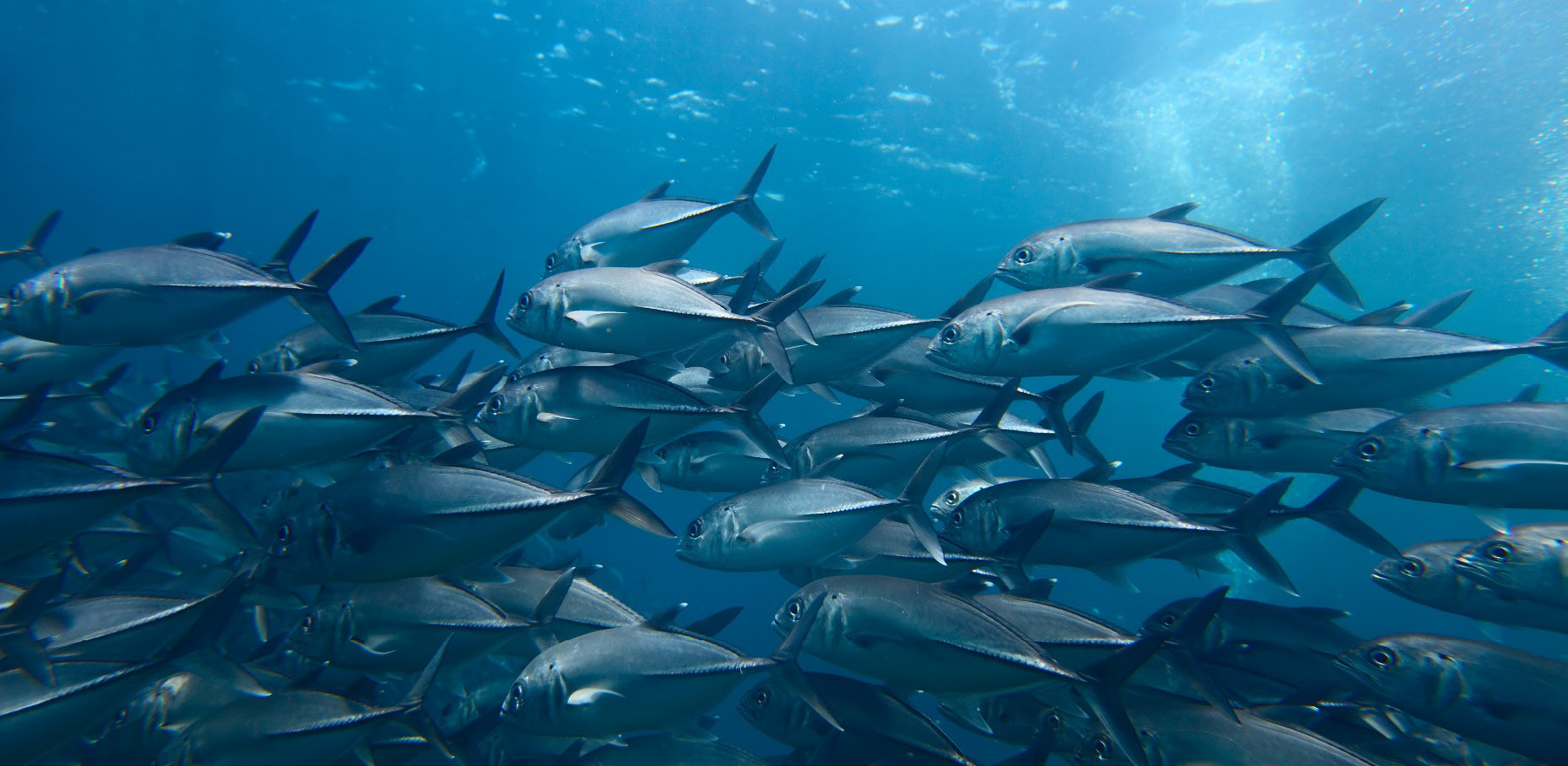
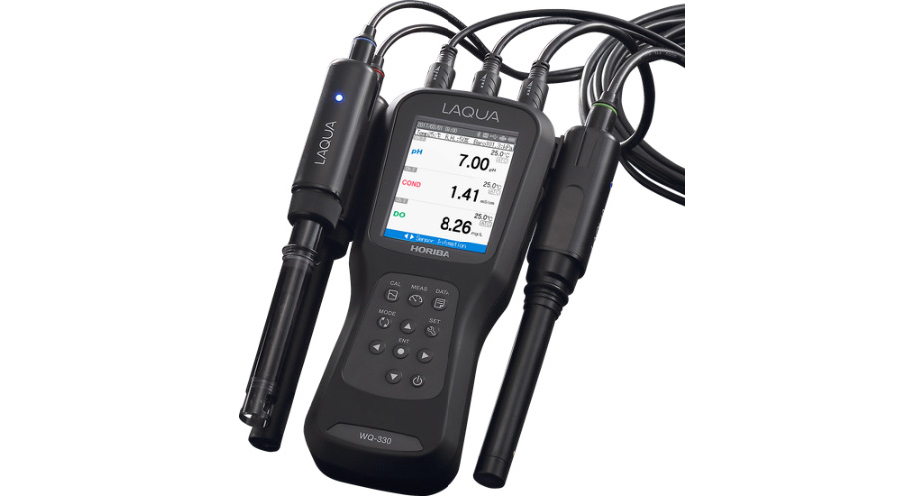
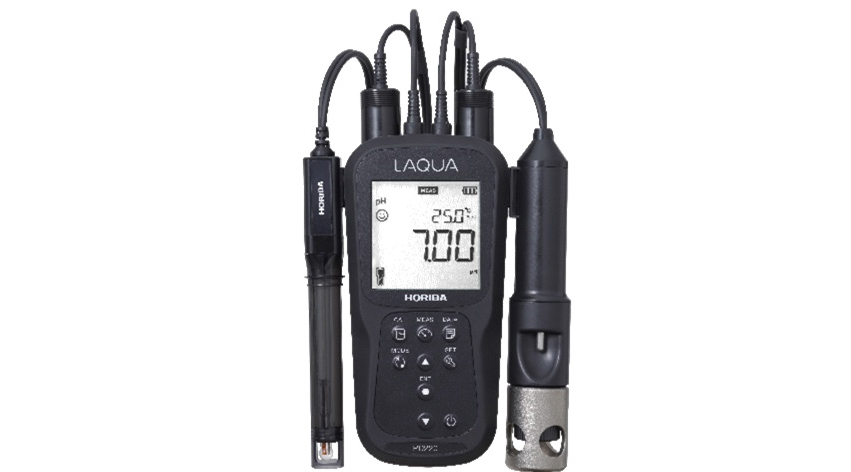
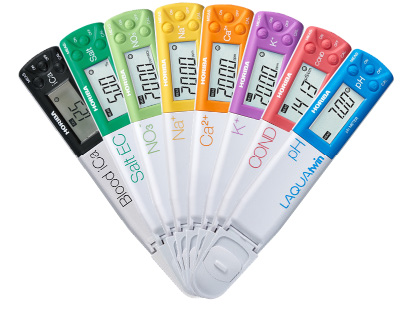
Parameters in Aquaculture
In aquaculture, portable instruments are used to measure several critical water quality parameters — not just dissolved oxygen — to keep aquatic animals healthy and productive. Here are the main parameters typically measured with portable instruments:
- Dissolved Oxygen (DO)
- Most critical; fish and shrimp require specific DO levels.
- Low DO can cause stress, disease, or death.
- Temperature
- Water temperature affects metabolism, oxygen solubility, and feeding rates.
- Some species have narrow optimal temperature ranges.
- pH
- Measures acidity/basicity of the water.
- pH affects ammonia toxicity and overall fish health.
- Salinity (or Conductivity)
- Important for species in brackish or marine environments.
- Helps maintain osmotic balance in aquatic animals.
- Ammonia (NH₃/NH₄⁺)
- Toxic waste product from animal metabolism and decomposition.
- Even low concentrations can be deadly.
- Nitrite (NO₂⁻) and Nitrate (NO₃⁻)
- By-products of the nitrogen cycle.
- High nitrite is highly toxic; high nitrate can cause long-term health issues.
- Turbidity
- Measures water clarity.
- High turbidity can stress fish, clog gills, and block light for aquatic plants.
- Carbon Dioxide (CO₂)
- High CO₂ can lower blood pH in fish (acidosis).
- Important in systems with high stocking densities.
- Alkalinity
- Acts as a buffer against pH changes.
- Helps stabilize water chemistry.
- Chlorine
- Especially important when using treated tap water.
- Even small amounts can be harmful to aquatic life.
- ORP
- ORP is like a "health meter" for aquaculture water — it helps you catch problems early (like dirty water, low oxygen, or bad bacteria) before they stress or kill your animals.
Sources and references:
- Boyd, C. E., & Tucker, C. S. (1998). Pond Aquaculture Water Quality Management. Springer.
- Timmons, M. B., & Ebeling, J. M. (2013). Recirculating Aquaculture Systems. Cayuga Aqua Ventures.
- Hargreaves, J. A., & Tucker, C. S. (2004). Managing Salinity in Pond Aquaculture. Southern Regional Aquaculture Center, SRAC Publication No. 4603.
- FAO (Food and Agriculture Organization). Water Quality in Freshwater Fish Culture. FAO Fisheries and Aquaculture Technical Papers.
- Liao, I. C., & Chien, Y. H. (2011). Effects of salinity on aquaculture species. Aquaculture Research, 42(3), 293–298
Relationships Between Water Parameters
The different water quality parameters in aquaculture are closely interconnected, and changes in one often affect the others.
Parameter | Influences | Result |
---|---|---|
pH | NH₃ | When pH is higher than 9, ammonium converts to ammonia |
NH₄⁺ | When pH is lower than 9, ammonia converts to ammonium | |
Temperature | pH | Higher temperature can slightly increase pH because CO₂ solubility drops |
DO | Higher water temperatures reduce the amount of oxygen that water can hold | |
NH₃ | Higher temperature increases the pH, this can convert NH₄⁺ to NH₃ | |
Turbidity | DO | High turbidity reduces light penetration, reducing photosynthesis by aquatic plants and algae, which lowers DO production during the day |
CO₂ | pH | More CO₂ in water lowers pH (makes it more acidic) |
DO | High CO₂ levels can depress oxygen availability to fish |
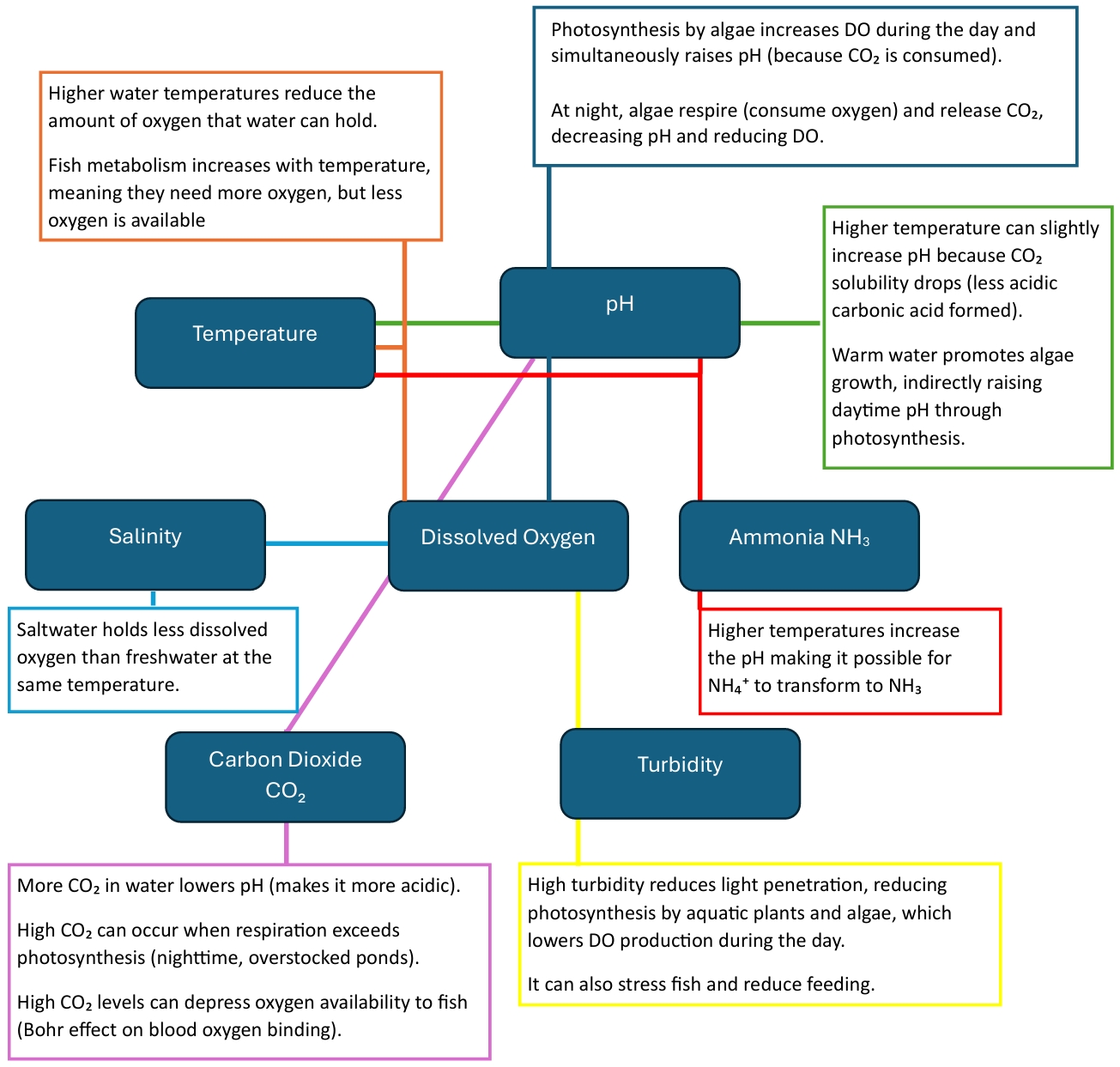
The Importance of Dissolved Oxygen in Aquaculture Farming
Dissolved oxygen (DO) is one of the most critical water quality parameters in aquaculture, directly impacting the survival, growth, and health of aquatic organisms. Fish, shrimp, and other farmed species rely on oxygen dissolved in water for respiration. Insufficient DO levels can lead to stress, reduced feeding, slower growth, increased disease susceptibility, and ultimately, high mortality rates (Boyd, 1998).
Sources such as Boyd and Tucker (2012) emphasize that optimal DO concentrations should typically remain above 5 mg/L for most species to thrive. Levels below 3 mg/L can cause severe stress, while prolonged exposure to low oxygen can trigger fish kills. Furthermore, low DO can impair the function of beneficial nitrifying bacteria, slowing the breakdown of harmful ammonia and nitrites in the water (Timmons & Ebeling, 2010).
Several factors influence DO levels, including temperature, salinity, organic load, and photosynthetic activity. Warmer water holds less oxygen, and biological activities like decomposition consume oxygen, particularly at night when photosynthesis ceases. Thus, maintaining adequate aeration through mechanical devices (e.g., paddle wheels, air stones) is essential, especially in intensive aquaculture systems.
Monitoring dissolved oxygen is not only critical for animal welfare but also for farm profitability and sustainability. Regular measurements allow early detection of problems, preventing catastrophic losses and ensuring consistent production. As aquaculture continues to expand globally, careful management of DO remains a cornerstone of responsible and successful farming practices.
Species | Ideal DO Level | Critical DO Level (Stress/Risk Zone) |
Notes |
---|---|---|---|
Tilapia (Oreochromis spp.) | 4.0–6.0 mg/L | <2.0 mg/L | Tilapia are fairly tolerant but do best with moderate oxygen levels. Below 2.0 mg/L, growth and immune function are significantly impaired. |
Channel Catfish (Ictalurus punctatus) | 4.0–6.0 mg/L | <2.0 mg/L | Catfish are resilient but need sufficient oxygen for growth. Low DO (<2.0 mg/L) reduces feeding efficiency and increases stress. |
Common Carp (Cyprinus carpio) | 4.0–6.0 mg/L | <2.0 mg/L | Carp can handle lower oxygen levels but growth and reproduction are limited below 3.0 mg/L. Below 2.0 mg/L, fish become lethargic. |
Rainbow Trout (Oncorhynchus mykiss) | 8.0–10.0 mg/L | <4.0 mg/L | Trout are highly sensitive to low DO levels. Anything below 4.0 mg/L can cause stress, poor feeding, and health decline. |
Atlantic Salmon (Salmo salar) | 8.0–10.0 mg/L | <4.0 mg/L | Salmon require high DO levels to thrive. Below 4.0 mg/L, growth rates slow, and the fish are more susceptible to diseases. |
Shrimp (Litopenaeus vannamei) | 4.0–6.0 mg/L | <2.0 mg/L | Shrimp can tolerate some low DO, but poor oxygenation affects molting and survival. Levels <2.0 mg/L increase susceptibility to bacterial infections. |
Barramundi (Lates calcarifer) | 5.0–7.0 mg/L | <3.0 mg/L | Barramundi prefer moderate oxygen levels. Low DO (<3.0 mg/L) causes weakness, poor appetite, and increased stress. |
Asian Seabass (Lates calcarifer) | 5.0–7.0 mg/L | <3.0 mg/L | Like barramundi, they thrive with moderate DO levels. Below 3.0 mg/L, fish exhibit stress responses and decreased growth. |
Koi Carp (Cyprinus carpio koi) | 5.0–7.0 mg/L | <3.0 mg/L | Koi require oxygen to maintain color vibrancy and overall health. Low DO (<3.0 mg/L) leads to weakness and poor coloration. |
The Importance of Temperature in Aquaculture Farming
Temperature is one of the most important environmental factors in aquaculture, influencing almost every aspect of aquatic life, from metabolism and growth to immune function and reproduction. Each species has an optimal temperature range where it thrives; deviations can cause stress, slow growth, and increase disease susceptibility (Boyd, 1998).
According to Timmons and Ebeling (2010), temperature affects oxygen solubility in water—warmer water holds less dissolved oxygen, compounding stress for farmed species. Furthermore, feeding rates, digestion, and nutrient assimilation are all temperature-dependent. For instance, tilapia grow best at 27–30°C, while rainbow trout require cooler waters around 12–18°C. Exposure to temperatures outside of these ranges can impair feeding efficiency, lower resistance to infections, and, at extreme levels, cause mortality.
Temperature also directly impacts the rate of ammonia toxicity. As water temperature rises, the toxic form of ammonia (NH₃) becomes more prevalent, increasing the risk to aquatic organisms (Boyd & Tucker, 2012). Therefore, maintaining stable and species-appropriate temperatures is critical not just for growth performance but also for overall water quality management.
Proper temperature control is essential in intensive aquaculture systems like recirculating aquaculture systems (RAS), where environmental conditions must be tightly managed to maximize productivity and animal welfare. Tools such as heating systems, shading, and aeration are often used to mitigate temperature fluctuations and ensure stable, optimal conditions year-round.
Species | Ideal Temperature Range | Critical Temperature (Stress/Risk Zone) |
Notes |
---|---|---|---|
Tilapia (Oreochromis spp.) | 25–30°C | <18°C or >35°C | Tilapia are warm-water fish. Below 18°C, their growth slows, and below 12°C, they can suffer from stress and disease. Above 35°C, they experience heat stress. |
Channel Catfish (Ictalurus punctatus) | 20–30°C | <15°C or >35°C | Catfish do well in moderate temperatures. Below 15°C, they stop feeding, and above 35°C, they become stressed and prone to oxygen depletion. |
Common Carp (Cyprinus carpio) | 20–28°C | <10°C or >32°C | Carp are tolerant but thrive between 20–28°C. Below 10°C, their metabolism slows, and above 32°C, they risk oxygen stress and reduced growth. |
Rainbow Trout (Oncorhynchus mykiss) | 12–18°C | <8°C or >22°C | Trout are cold-water species. Below 8°C, they become lethargic, and above 22°C, growth slows and health declines. |
Atlantic Salmon (Salmo salar) | 8–18°C | <4°C or >22°C | Salmon are sensitive to warm water. Below 4°C, their metabolism decreases, and above 22°C, they experience heat stress and reduced feed conversion. |
Shrimp (Litopenaeus vannamei) | 28–30°C | <22°C or >34°C | Shrimp prefer warm water. Below 22°C, they become stressed, and above 34°C, molting issues and disease risks increase. |
Barramundi (Lates calcarifer) | 25–30°C | <20°C or >35°C | Barramundi thrive in moderate warmth. Below 20°C, their immune system weakens, and above 35°C, they experience heat stress and slow growth. |
Asian Seabass (Lates calcarifer) | 25–30°C | <20°C or >35°C | Similar to barramundi, they prefer warmer water but suffer from heat stress above 35°C and slow metabolism below 20°C. |
Koi Carp (Cyprinus carpio koi) | 18–24°C | <10°C or >30°C | Koi are temperate fish. Below 10°C, they become lethargic, and above 30°C, their immune system weakens, and they are prone to diseases. |
The Importance of pH in Aquaculture Farming
pH is a crucial water quality parameter in aquaculture, reflecting the balance between acidity and alkalinity in the water. It directly affects the physiological health of farmed species, the efficiency of biological filtration, and the overall stability of the aquatic environment (Boyd, 1998).
Most aquaculture species, such as tilapia, catfish, and shrimp, perform best within a relatively narrow pH range, typically between 6.5 and 8.5 (Boyd & Tucker, 2012). When pH falls outside of this range, it can impair respiration, reduce feeding rates, and weaken immune systems. For example, acidic conditions (pH below 6.0) can damage fish gills and limit oxygen uptake, while overly alkaline conditions (pH above 9.0) can increase the toxicity of ammonia (Timmons & Ebeling, 2010).
The relationship between pH and ammonia toxicity is particularly critical. At higher pH levels, a greater proportion of total ammonia exists in its toxic un-ionized form (NH₃), posing a greater threat to aquatic life. Similarly, low pH can hinder the activity of nitrifying bacteria responsible for converting ammonia into less harmful compounds, disrupting biofiltration and leading to water quality problems.
Natural factors like photosynthesis and respiration cause daily fluctuations in pH, especially in ponds rich with algae. Therefore, regular pH monitoring is essential to prevent harmful swings and ensure optimal growing conditions. Buffering the water with materials like agricultural lime can help stabilize pH and maintain a safe environment for the cultured species.
In short, maintaining a stable, appropriate pH is fundamental for maximizing health, growth, and production efficiency in aquaculture systems.
Species | Ideal pH Range | Critical pH (Stress/Risk Zone) |
Notes |
---|---|---|---|
Tilapia (Oreochromis spp.) | 6.5 – 8.5 | <6.0 or >9.0 | Highly tolerant, but sudden drops below 6.0 cause stress and disease vulnerability. |
Channel Catfish (Ictalurus punctatus) | 6.5 – 8.5 | <5.5 or >9.5 | Resilient species, but gill damage and feeding reduction occur at critical extremes. |
Common Carp (Cyprinus carpio) | 6.5 – 8.5 | <5.5 or >9.5 | Carp are hardy but growth slows if pH stays outside the ideal range for long. |
Rainbow Trout (Oncorhynchus mykiss) | 6.5 – 7.5 | <6.0 or >8.0 | Sensitive species; low pH (<6.0) severely affects respiration and survival. |
Atlantic Salmon (Salmo salar) | 6.5 – 7.5 | <6.0 or >8.0 | Optimal performance within narrow pH; prone to skin and gill damage at low pH. |
Shrimp (Litopenaeus vannamei) | 7.5 – 8.5 | <7.0 or >9.0 | Shrimp require slightly alkaline water; acidic conditions weaken shell and immune system. |
Barramundi (Lates calcarifer) | 7.0 – 8.5 | <6.5 or >9.0 | Can tolerate slight variation but extreme low or high pH increases mortality risk. |
Asian Seabass (Lates calcarifer) | 7.0 – 8.5 | <6.5 or >9.0 | Needs stable pH; fluctuating conditions harm digestion and osmoregulation. |
Koi Carp (Cyprinus carpio koi) | 7.0 – 8.5 | <6.0 or >9.0 | Display color loss, stress, and susceptibility to parasites if pH strays too far. |
The Importance of Salinity in Aquaculture Farming
Salinity, the concentration of dissolved salts in water, is a critical environmental factor in aquaculture. It directly affects fish health, growth, reproduction, and system stability. Marine, brackish, and freshwater species each have different salinity requirements. Managing salinity effectively supports optimal physiological performance and reduces stress and disease risk.
Each species has a preferred salinity range. Marine organisms like shrimp (Litopenaeus vannamei) thrive at 15–35 ppt, while freshwater species such as tilapia (Oreochromis spp.) prefer <5 ppt. Brackish species, like milkfish, tolerate intermediate levels. Matching species with proper salinity is essential for efficient production.
Salinity affects the balance of salts and water within aquatic animals. Deviations from the optimal range force organisms to expend energy on osmoregulation, reducing growth and weakening immunity. Stable, species-specific salinity levels help ensure better survival and reduced disease outbreaks.
Salinity also influences water chemistry, including pH, oxygen levels, and the toxicity of ammonia and metals. At higher salinities, toxic ammonia is more likely to convert to its less harmful form. Salinity also impacts mineral availability and gas solubility, making it a vital component of broader water quality management.
In ponds, salinity is affected by water source, rainfall, and evaporation. In recirculating aquaculture systems (RAS), it must be carefully maintained due to minimal water exchange. Continuous monitoring and periodic adjustments, using salt or freshwater, help keep levels stable.
Salinity plays a central role in aquaculture health and productivity. Monitoring and maintaining optimal levels tailored to the species and system type supports efficient growth, reduces stress, and improves overall farm outcomes.
Species | Ideal Salinity Range | Critical Salinity (Stress/Risk Zone) |
Notes |
---|---|---|---|
Tilapia (Oreochromis spp.) | 0 – 10 ppt | >20 ppt (depending on strain) | Primarily freshwater, but some strains tolerate mild brackish water; high salinity stresses osmoregulation. |
Channel Catfish (Ictalurus punctatus) | 0 – 5 ppt | >10 ppt | Freshwater species; salinity >10 ppt causes stress, poor growth, and mortality. |
Common Carp (Cyprinus carpio) | 0 – 5 ppt | >10 ppt | Tolerates low brackish water but growth slows beyond 5–8 ppt; prolonged exposure harms health. |
Rainbow Trout (Oncorhynchus mykiss) | 0 – 8 ppt (for freshwater farming) | >10–12 ppt | Freshwater-adapted; anadromous strains tolerate up to 30 ppt after gradual acclimation. |
Atlantic Salmon (Salmo salar) | 0 (juvenile) → 30–35 ppt (adult) | Rapid change >5 ppt/day | Juveniles (parr, smolt) need gradual acclimation to seawater; adults thrive in marine salinity. |
Shrimp (Litopenaeus vannamei) | 5 – 35 ppt | <2 ppt or sudden changes >5 ppt | Very euryhaline; can survive from brackish to full seawater, but sudden shifts are dangerous. |
Barramundi (Lates calcarifer) | 1 – 30 ppt | Rapid salinity swings >10 ppt/day | Euryhaline species; thrives in both freshwater and seawater with gradual acclimation. |
Asian Seabass (Lates calcarifer) | 1 – 30 ppt | Rapid salinity swings >10 ppt/day | Needs stable salinity; sudden drops cause osmoregulatory shock. |
Koi Carp (Cyprinus carpio koi) | 0 – 5 ppt (for general health) | >8–10 ppt | Freshwater ornamental species; salinity above 8 ppt stresses metabolism and reduces vitality. |
The Importance of Ammonium in Aquaculture Farming
Ammonia management is critical in aquaculture because ammonia, a waste product excreted by fish and resulting from decomposing organic matter, is highly toxic even at low concentrations. In water, ammonia exists in two forms: un-ionized ammonia (NH₃), which is highly toxic, and ionized ammonium (NH₄⁺), which is relatively non-toxic. The balance between these forms depends on water temperature and pH—higher temperatures and higher pH favor the more toxic un-ionized form (Boyd, 1998).
High levels of ammonia can cause serious health problems for aquatic species, including gill damage, reduced oxygen uptake, impaired growth, suppressed immune function, and ultimately death (Boyd & Tucker, 2012). Stress from ammonia toxicity often makes fish and shrimp more vulnerable to bacterial and parasitic infections. Chronic exposure to even low levels of ammonia can stunt growth and reduce feed conversion efficiency, leading to economic losses.
In well-managed aquaculture systems, biological filtration—through nitrifying bacteria—converts toxic ammonia into nitrite and then into relatively harmless nitrate. However, if the system is overloaded with waste (from overfeeding or high stocking densities) or if water temperature and pH conditions change, ammonia can quickly accumulate to dangerous levels (Timmons & Ebeling, 2010).
Regular monitoring of total ammonia nitrogen (TAN) and maintaining favorable pH and temperature conditions are essential strategies for preventing ammonia toxicity. Good practices such as controlled feeding, maintaining healthy biofilters, and regular water exchanges are also critical for keeping ammonia at safe levels.
Proper ammonia management not only ensures the health and growth of aquaculture species but is fundamental to sustainable and profitable farming operations.
Species | Ideal Ammonium (NH₄⁺) Level | Critical NH₄⁺ (Stress/Risk Zone) |
Notes |
---|---|---|---|
Tilapia (Oreochromis spp.) | <1.0 mg/L | >3.0 mg/L | Tolerant to some ammonium, but long exposure >3.0 mg/L stresses kidneys and reduces feed intake. |
Channel Catfish (Ictalurus punctatus) | <1.0 mg/L | >2.5 mg/L | Ammonium buildup lowers dissolved oxygen indirectly by encouraging algal growth and decomposition. |
Common Carp (Cyprinus carpio) | <1.0 mg/L | >2.0 mg/L | Prolonged high NH₄⁺ damages gill structure and inhibits ammonia excretion, causing stress. |
Rainbow Trout (Oncorhynchus mykiss) | <0.5 mg/L | >1.0 mg/L | Sensitive species; even "non-toxic" ammonium at high levels (>1 mg/L) stresses respiration. |
Atlantic Salmon (Salmo salar) | <0.5 mg/L | >1.0 mg/L | Juveniles especially vulnerable; NH₄⁺ interferes with blood ion balance (osmoregulation). |
Shrimp (Litopenaeus vannamei) | <1.0 mg/L | >2.5 mg/L | Shrimp can tolerate moderate ammonium, but prolonged exposure reduces molting and survival rates. |
Barramundi (Lates calcarifer) | <1.0 mg/L | >2.0 mg/L | Moderate tolerance; excess ammonium reduces appetite and increases susceptibility to disease. |
Asian Seabass (Lates calcarifer) | <1.0 mg/L | >2.0 mg/L | Requires careful monitoring especially in intensive systems to avoid chronic stress. |
Koi Carp (Cyprinus carpio koi) | <1.0 mg/L | >2.0 mg/L | Chronic high ammonium exposure dulls color vibrancy and causes poor growth. |
The Importance of Nitrate in Aquaculture Farming
Nitrate (NO₃⁻) is the final product of the nitrogen cycle in aquaculture systems, resulting from the bacterial oxidation of nitrite. While nitrate is significantly less toxic than ammonia or nitrite, high concentrations over time can still negatively affect aquatic species, particularly in closed and recirculating aquaculture systems (RAS) (Boyd, 1998).
At moderate levels, nitrate is generally tolerated by most fish and shrimp. However, chronic exposure to elevated nitrate levels (typically above 80–100 mg/L for many freshwater species) can cause stress, reduce growth rates, impair reproductive performance, and weaken immune function (Timmons & Ebeling, 2010). Some sensitive species, like marine fish and ornamental species, are even more vulnerable to nitrate accumulation.
Unlike ammonia and nitrite, nitrate does not easily volatilize or break down in water, making its removal more challenging. Methods to control nitrate include water exchange, the use of denitrification systems (which convert nitrate to nitrogen gas under anaerobic conditions), and the integration of plants or algae that uptake nitrate (Boyd & Tucker, 2012).
In well-managed systems, keeping nitrate concentrations low helps maintain optimal fish health, supports better water quality, and enhances sustainability by reducing the need for frequent water replacement. Monitoring nitrate levels regularly is especially important in intensive and high-density farming operations.
While less immediately dangerous than ammonia or nitrite, managing nitrate effectively is crucial for the long-term health and success of aquaculture farms.
Species | Ideal Nitrate Level | Critical Nitrate (Stress/Risk Zone) |
Notes |
---|---|---|---|
Tilapia (Oreochromis spp.) | <50 mg/L | >200 mg/L | Tilapia tolerate moderate nitrate well, but high levels (>200 mg/L) stress kidneys and reduce growth. |
Channel Catfish (Ictalurus punctatus) | <50 mg/L | >150 mg/L | Resilient species, but chronic exposure >150 mg/L increases disease outbreaks. |
Common Carp (Cyprinus carpio) | <50 mg/L | >150 mg/L | Good tolerance but prolonged high nitrate affects reproductive health and egg quality. |
Rainbow Trout (Oncorhynchus mykiss) | <10 mg/L | >50 mg/L | Very sensitive; nitrate >50 mg/L impacts gill function and growth severely. |
Atlantic Salmon (Salmo salar) | <10 mg/L | >50 mg/L | Juveniles are especially vulnerable; nitrate >50 mg/L lowers survival rates. |
Shrimp (Litopenaeus vannamei) | <50 mg/L | >100 mg/L | Tolerates moderate nitrate, but long exposure above 100 mg/L reduces molting and immune defense. |
Barramundi (Lates calcarifer) | <50 mg/L | >100 mg/L | Moderate tolerance; elevated nitrate reduces feed efficiency and weight gain. |
Asian Seabass (Lates calcarifer) | <50 mg/L | >100 mg/L | Chronic nitrate stress weakens osmoregulation and liver function. |
Koi Carp (Cyprinus carpio koi) | <50 mg/L | >100 mg/L | High nitrate dulls colors, stunts growth, and makes koi prone to skin infections. |
The Importance of Turbidity in Aquaculture Farming
Turbidity refers to the cloudiness or haziness of water caused by suspended particles such as clay, organic matter, plankton, and uneaten feed. In aquaculture, managing turbidity is important because it affects light penetration, water chemistry, and the health of aquatic species (Boyd, 1998).
Moderate turbidity can be beneficial by providing shade, reducing excessive algal growth, and offering some protection to fish from predators. However, high turbidity—especially from inorganic particles like clay—can cause serious problems. It can clog fish gills, reduce feeding efficiency, stress the animals, and promote the spread of pathogens (Boyd & Tucker, 2012). In addition, excessive turbidity can limit photosynthesis by phytoplankton, which may lead to lower dissolved oxygen production and unstable water conditions.
Organic turbidity, caused by uneaten feed, waste, and decaying organisms, is particularly dangerous. It fuels bacterial growth, leading to oxygen depletion and the buildup of harmful substances like ammonia and nitrite (Timmons & Ebeling, 2010).
Managing turbidity involves practices such as minimizing erosion around ponds, controlling feeding rates, using sedimentation basins, and applying flocculants or coagulants if necessary. Regular monitoring helps ensure that turbidity levels stay within safe ranges for the cultured species—typically less than 25–50 NTU (Nephelometric Turbidity Units) for clear-water species like trout, while more tolerant species like catfish can handle higher levels.
In short, controlling turbidity is crucial not only for maintaining good water quality but also for promoting healthy, productive aquaculture operations.
Species | Ideal Turbidity Range | Critical Turbidity (Stress/Risk Zone) |
Notes |
---|---|---|---|
Tilapia (Oreochromis spp.) | 30–80 NTU | <10 NTU or >150 NTU | Tilapia tolerate moderate turbidity well; very clear water (<10 NTU) increases predation stress; very muddy water (>150 NTU) clogs gills. |
Channel Catfish (Ictalurus punctatus) | 30–100 NTU | <20 NTU or >150 NTU | Prefer slightly turbid water; high turbidity (>150 NTU) reduces oxygen exchange at gills and affects feeding. |
Common Carp (Cyprinus carpio) | 30–80 NTU | <10 NTU or >120 NTU | Carp do well in moderately turbid ponds; excessive turbidity (>120 NTU) affects filter feeding and health. |
Rainbow Trout (Oncorhynchus mykiss) | 5–20 NTU | >25 NTU | Trout need clear water; turbidity >25 NTU stresses gills, reduces visibility, and suppresses feeding. |
Atlantic Salmon (Salmo salar) | 5–20 NTU | >25 NTU | Salmon also require clear water; chronic turbidity causes gill hyperplasia and stress. |
Shrimp (Litopenaeus vannamei) | 20–80 NTU | <10 NTU or >150 NTU | Shrimp tolerate a broad range, but heavy solids (>150 NTU) block gill function and settle in gill chambers. |
Barramundi (Lates calcarifer) | 20–80 NTU | >120 NTU | Moderate turbidity is acceptable; very high turbidity reduces prey visibility and can stress juveniles. |
Asian Seabass (Lates calcarifer) | 20–80 NTU | >120 NTU | Similar to barramundi; critical to keep balanced during larval stages to prevent feeding problems. |
Koi Carp (Cyprinus carpio koi) | 10–40 NTU | >80 NTU | Ornamental fish need relatively clear water; high turbidity (>80 NTU) hides colors and stresses skin/mucus layers. |
The Importance of ORP in Aquaculture Farming
Oxidation-Reduction Potential (ORP) measures the ability of water to either release or accept electrons during chemical reactions, serving as an indicator of water quality and overall system health. In aquaculture, ORP is particularly important because it reflects the balance between oxidizing agents (like oxygen) and reducing agents (like organic waste) in the water (Boyd, 1998).
An optimal ORP level, typically between +200 and +300 millivolts (mV), indicates good water quality with sufficient oxygen to support healthy aquatic life (Boyd & Tucker, 2012). When ORP values drop too low (below +150 mV), it suggests poor water quality, a buildup of organic waste, low dissolved oxygen, and potentially toxic conditions such as elevated ammonia or hydrogen sulfide. This environment can stress fish and shrimp, impair their immune systems, and increase mortality rates.
On the other hand, very high ORP levels (above +400 mV), especially when strong oxidizing agents like ozone are used, can also be harmful. Over-oxidation can damage fish gill tissues and lead to oxidative stress (Timmons & Ebeling, 2010).
ORP is especially valuable in intensive systems like recirculating aquaculture systems (RAS), where water quality can change rapidly. Monitoring ORP helps farmers detect early signs of water quality deterioration or biological filter malfunctions before they become visible through other parameters. It can also guide the controlled use of ozone for disinfection without harming the stock.
In summary, maintaining an optimal ORP range is essential for ensuring a healthy, stable environment, maximizing growth, and preventing disease outbreaks in aquaculture farming.
Species | Ideal ORP Range | Critical ORP (Stress/Risk Zone) |
Notes |
---|---|---|---|
Tilapia (Oreochromis spp.) | +150 to +250 mV | <+100 mV | Tilapia thrive in water with good oxygenation and biological activity; low ORP (<+100 mV) indicates poor water quality and potential anaerobic zones. |
Channel Catfish (Ictalurus punctatus) | +150 to +250 mV | <+100 mV | Channel catfish prefer higher ORP, indicating efficient biological filtration and oxygen availability. Low ORP stresses fish and promotes disease. |
Common Carp (Cyprinus carpio) | +150 to +250 mV | <+100 mV | Carp tolerate a moderate ORP range, but low ORP (<+100 mV) can impair immune function and growth. |
Rainbow Trout (Oncorhynchus mykiss) | +200 to +350 mV | <+150 mV | Trout require high ORP (healthy biofilm, good oxygenation) to avoid stress, especially in recirculating systems. ORP below +150 mV indicates poor water quality. |
Atlantic Salmon (Salmo salar) | +200 to +350 mV | <+150 mV | Salmon, like trout, need a higher ORP to maintain health; low ORP (<+150 mV) results in reduced oxygen and increased risk of bacterial infections. |
Shrimp (Litopenaeus vannamei) | +150 to +250 mV | <+100 mV | Shrimp are sensitive to low ORP levels; poor oxygenation and anaerobic conditions (<+100 mV) impair molting, growth, and survival. |
Barramundi (Lates calcarifer) | +150 to +250 mV | <+100 mV | Barramundi require a good balance of oxygen; low ORP (<+100 mV) stresses fish, leading to poor feeding and immune function. |
Asian Seabass (Lates calcarifer) | +150 to +250 mV | <+100 mV | Similar to barramundi, low ORP (<+100 mV) causes sluggish behavior, reduced feeding, and disease susceptibility. |
Koi Carp (Cyprinus carpio koi) | +150 to +250 mV | <+100 mV | Ornamental koi require good water quality to maintain color and health; low ORP (<+100 mV) leads to poor water quality and health deterioration. |
The HORIBA Measurement Solutions
With over 75 years of engineering excellence, HORIBA's innovative and diverse range of water quality analyzers, electrodes and solutions are ideal for everyday needs in the laboratory and field through to the most demanding of applications.
HORIBA LAQUAtwin Pocket Meter Series
The LAQUAtwin pocket meters are compact, lightweight, and highly precise. The series features 15 models covering 10 key parameters, including pH, conductivity, ORP, nitrate, and salinity. These affordable, single-parameter devices are IP67 waterproof and provide fast, reliable water quality measurements on the go.
HORIBA LAQUA 200 Series Portable Meters
The LAQUA 200 Series features a selection of 10 IP67 waterproof meters designed for measuring pH, ORP, conductivity, salinity, and dissolved oxygen. Available in single and dual-parameter models, the series includes two product lines: the 210, capable of storing up to 500 data sets, and the 220, which holds up to 1000 data sets and offers PC connectivity. Dissolved oxygen measurements are performed using a reliable galvanic sensor.
HORIBA LAQUA 300 Series Portable Meters
The LAQUA 300 Series includes three advanced digital meters, with the WQ300 capable of measuring up to three parameters simultaneously in any combination. Supported parameters include pH, ORP, conductivity, salinity, ions, and dissolved oxygen (using an optical probe). The smart electrodes feature built-in memory to store calibration data, allowing seamless transfer between meters without the need for re-calibration.
HORIBA U-50 Series Portable Meters
The U-50 Series is a multi-parameter sonde instrument featuring integrated sensors for pH, ORP, conductivity, salinity, dissolved oxygen, and turbidity—all contained within a single IP68 waterproof housing. The series offers 20 models with various configurations, including options for depth measurement, extended cable lengths, and GPS positioning. Dissolved oxygen is measured using a durable polarographic sensor.
Product | pH | ORP | DO | Temp | Salinity | NH₃ | NH₄⁺ | NO₃⁻ | Turbidity |
---|---|---|---|---|---|---|---|---|---|
LAQUAtwin PH11, PH22, PH33 | ✓ | ✓ | |||||||
LAQUAtwin Salt11, Salt22 | ✓ | ✓ | |||||||
LAQUAtwin NO3-11 | ✓ | ✓ | |||||||
LAQUAtwin ORP-11 | ✓ | ✓ | |||||||
PH210, PH220 | ✓ | ✓ | ✓ | ✓ | |||||
PC210, PC220 | ✓ | ✓ | ✓ | ✓ | ✓ | ||||
PD210, PD220 | ✓ | ✓ | ✓ | ✓ | |||||
WQ310, WQ320, WQ330 | ✓ | ✓ | ✓ | ✓ | ✓ | ✓ | ✓ | ✓ | ✓ |
U-51 | ✓ | ✓ | ✓ | ✓ | ✓ | ||||
U-52, U-53, U-54 | ✓ | ✓ | ✓ | ✓ | ✓ | ✓ | |||
TB220 | ✓ |